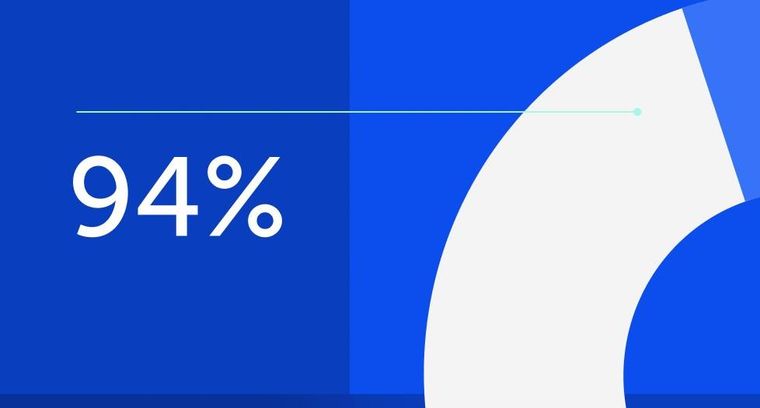
94% of researchers rate our articles as excellent or good
Learn more about the work of our research integrity team to safeguard the quality of each article we publish.
Find out more
ORIGINAL RESEARCH article
Front. Bee Sci., 10 April 2025
Sec. Bee Protection and Health
Volume 3 - 2025 | https://doi.org/10.3389/frbee.2025.1550560
Introduction: Bumblebees are essential pollinators in temperate regions of the northern hemisphere. Niche overlap and competition with honeybees may impose significant stress on bumblebees by reducing nutrient acquisition and increasing the risk of pathogen and parasite spillover from honeybees. One of these parasites is the bumblebee wax moth (Aphomia sociella), whose larvae can be found in bumblebee and wasp nests, as well as weakened commercial honeybee hives (hereafter called apiaries).
Methods: Using Bombus terrestris colonies as experimental models, we expected that young queen and worker bumblebee immunity (measured by encapsulation response) would become weaker under both competitive (i.e., proximity to apiaries) and parasite (A. sociella infestation) pressure and, specifically, that the immunity of bumblebees in closest proximity to apiaries would be weakest in nests infested by A. sociella.
Results and discussion: We observed increased infestation, lower reproductive output, and weaker encapsulation response in bumblebee colonies that were near apiaries. Our data provide insights on the ecology of A. sociella infestations where honeybees and bumblebees coexist. Our observations of reduced immune response in bumblebees inhabiting colonies nearer to apiaries are of critical importance, as this reduction in immune response could facilitate additional infestations of other parasites and pathogens within bumblebee colonies.
Insects comprise over 80% of all animal species (Stork, 2018). They form a foundational part of trophic levels and dominate many of Earth’s ecosystems (Stork, 2018; Wilson and Fox, 2021). For example, more than 60% of bird species rely on insects as an adult food source, and many depend on insect food during ontogeny. However, more than half of Earth’s natural terrestrial ecosystems are currently under significant human pressure (Wagner et al., 2021), resulting in dramatic declines in insect numbers and diversity (Cardoso et al., 2020; Van Klink et al., 2020). Even protected areas in Germany have lost more than 75% of flying insect biomass (Hallmann et al., 2017). These negative trends in insect diversity, abundance, and ecological service provision are driven by factors such as habitat loss or fragmentation, climate change, invasive competitors, pathogens, parasites and predators, intensive agriculture, modern forestry measures, human-induced fires, environmental pollution, overexploitation of water resources, and pesticide use (Wagner, 2020; Dicks et al., 2021; Raven and Wagner, 2021).
A recent study shows that global production of essential foods is being limited by a lack of pollinators (Turo et al., 2024). Between 75–95% of the world’s flowering plant species sexually reproduce via pollination by animals (Potts et al., 2010; Ollerton et al., 2011). Animals pollinate over 180,000 plant species and over 1200 crop species, supporting approximately 35% of global crop production (Klein et al., 2007). Insects comprise most pollinator species, with bees, flies, butterflies and moths, wasps, beetles, and ants pollinating most wild and domesticated plants.
The European honeybee (Apis mellifera) is the most well-known honeybee species globally (Goulson et al., 2015). These domesticated bees are important agricultural pollinators and also produce honey and wax that humans gather and use. Although honeybees are important pollinators of agricultural and wild plants, they are not native to many areas (Schweiger et al., 2010). They cannot properly pollinate many plant species compared to co-evolved native pollinator insects. Bumblebees (Bombus spp.) are key native pollinators in temperate regions of the northern hemisphere (Fontaine et al., 2006). There is substantial evidence that many bumblebee species have declined throughout Europe, North America, South America, and Asia in recent decades due to habitat loss, lack of floral resources, novel introduced parasites (Schmid-Hempel et al., 2014; Salvarrey et al., 2021), or combinations of several stressors (Schweiger et al., 2010; Goulson et al., 2015; Martins et al., 2015; Evans et al., 2023).
Evidence suggests that competition with managed honeybees can negatively affect bumblebees. For example, Thomson (2004) found that foraging rates and reproductive success of bumblebee colonies were significantly reduced due to proximity to apiaries. Walther-Hellwig et al. (2006) reported that bumblebees avoided foraging at sites near apiaries. Some bumblebee species switched to foraging later in the day or changed foraging to less rewarding floral resources. These changes occurred because of significant overlap of foraging niches, often ranging between 80–90% between managed honeybees and native bumblebees (Thomson, 2006). Niche overlap and competition between species may have significant ecological consequences, as this can lower an organism’s fitness by reducing the amount of nutrient acquisition and increasing physiological stress (Page and Williams, 2023).
Another stressor in bumblebees is the spillover of pathogens and parasites from honeybees (Kosior et al., 2007; Szabo et al., 2012; Schmid-Hempel et al., 2014; Straub et al., 2022). In some cases, infections might also be transmitted from bumblebees to A. mellifera (Plischuk et al., 2011). The bumblebee wax moth (Aphomia sociella) is a small moth within Family Pyralidae and Subfamily Galleriinae. This parasite of social Hymenoptera is native to Europe, and its larvae are often found in bumblebee and wasp nests (Wallin et al., 2020). It can also invade weakened commercial honeybee colonies. A. sociella larvae inside honeybee colonies live behind a silk enclosure and are well protected from their hosts. At the beginning of the larval phase, the pest consumes the old wax cells, residue, pollen, nectar, and droppings of bumblebees. Large, final-instar larvae become predatory by attacking and consuming bumblebee larvae. A. sociella can occasionally be a pest of active honeybee colonies. However, the pest will usually take advantage of already diseased or declining honeybee colonies. In search of weakened honeybee colonies, the pest is attracted to apiaries and subsequently inspects its chance of invading the colony. This may make bumblebee nests near apiaries more vulnerable to A. sociella, and, thus, more susceptible to immunity and fitness complications.
In this study, we examined whether native bumblebees may simultaneously face both a higher probability of being invaded by A. sociella pests and severe interspecific competition from honeybees when their nests are in closer proximity to apiaries. First, we hypothesized that bumblebee immunity may become weaker due to competition. Proximity to honeybees can increase interspecific competition and parasite burden in bumblebee populations, and the highest likelihood of bumblebee nest infestations was expected near apiaries (Elbgami et al., 2014). Second, we predicted that parasite infestations also affect the immune response, such as encapsulation. During an encapsulation response against foreign bodies, the melanotic capsule, which consists of hemocytes, is formed (Gupta, 1986; Sadd and Schmid-Hempel, 2009). This capsule can block parasites’ absorption of nutrients and thus contribute to their killing by starvation (Strand, 2008) or the toxic effects of melanin production (Schmid-Hempel, 2021). We hypothesized that the immunity and reproductive output of bumblebees near apiaries would be weakest in bumblebee nests infested by A. sociella. We expected that the simultaneous effects of competition and infestation near apiaries might further decrease the strength of encapsulation response and the number of young queens and workers. In agricultural ecosystems, pest insects cause severe biotic stress and represent a significant constraint to crop production globally (Potts et al., 2016; Seibold et al., 2021; Chowdhury et al., 2023), and it is imperative that we devise and develop conservation plans to preserve biodiversity and maximize sustainable farming practices given the especially important roles of local pollinator populations (Orr et al., 2021; Russell et al., 2024).
Commercial B. terrestris colonies (Koppert Biological Systems, Berkel en Rodenrijs, Netherlands) were purchased and studied under field conditions. We used colonies originating from the same breeding line. We placed colonies near the town of Krāslava (55.9°N, 27.2°E) in southeast Latvia in mid-May of 2019 and 2024. Colonies were placed near six small apiaries containing 9–11 (mean = 10, SD = 0.63) honeybee colonies, and each colony produced between 350–650 queens, workers and drones. The study area is a mosaic of pine and alder forests, small plots of arable land, private gardens, and forest meadows. We never used the same apiary twice, and the minimum distance between the study sites (apiaries) was 5 km.
Bumblebee colonies were placed at five different distances from honeybee colonies: 0, 150, 300, 450, and 600 m. At each of these distances, five colonies were placed. Colonies at 0 m distance were placed in between honeybee colonies. The distance between bumblebee colonies was at least 4 m in each distance category.
When a colony was allocated to a study site, we removed the attached plastic container that had a feeding solution. Colonies were regularly checked to observe workers’ foraging flights, fanning behaviors, and colony sounds (Krams et al., 2021; Krama et al., 2022). We collected the colonies at the beginning of July as they reached the maximum of the young queen and male (generative offspring individuals) production phase. Twelve hours before final collection, we allowed all colony members to enter, but not exit, their respective colony. We then collected all colonies from each study site and placed them in a freezer (Angelantoni Life Science, Massa Martana, Italy) at −84 °C.
It is easy to detect the presence of A. sociella because the larvae spin strong silk to protect themselves while they feed. The silk is unmistakable, especially in the upper parts of the bumblebee hives. The silk layers are always very dense to shield the larvae from the hosts (Gambino, 1995). We regularly checked all bee and bumblebee colonies for the presence of A. sociella 2–3 times a week and measured its presence as binary (present or absent).
We checked for the number of hatched and unhatched worker and queen cells for each colony. We also counted the number of young queens (wings not worn) and workers, as these numbers reflect bumblebees’ reproductive effort (Krama et al., 2022).
We chose 6–9 worker bumblebees from each infested (n = 154 in total) and non-infested (n = 206 in total) colony and immobilized them on ice. We sterilized the abdomen of each bumblebee and punctured their cuticle by inserting a sterile needle between the third and fourth sternite (König and Schmid-Hempel, 1995; Schmid-Hempel, 1998). When the needle was removed, we inserted a piece of sterile nylon monofilament (3 mm length, 0.18 mm diameter, knotted at one end) into the puncture hole (Rantala et al., 2002). We let the nylon monofilament be exposed to the hemolymph for five hours, where it was encapsulated and melanized. A portion of the filament was left outside the bee for easy removal. We put the bumblebees in numbered plastic canisters (4 cm diameter, 8 cm height, covered with a mesh) and provided sugar water via moistened cotton. The canisters with bumblebees were placed in an incubator at a constant temperature of 24 °C.
The encapsulation response begins as soon as the cuticle of an insect is pierced, and the response usually lasts for hours (Dubovskii et al., 2010). Five hours after implantation, we carefully grasped the knot of the monofilament implant and removed the filament from each bumblebee. The removal of filaments after 5 h imitated the activity of the insect’s immune system in destroying or eliminating a parasite or its egg (Yourth et al., 2001, 2002). This time interval was chosen based on our preliminary studies which showed that 5 hours of exposure to the filament produces the most significant differences in encapsulation rate between individuals (Rantala et al., 2000), and more than 90% of individuals reach their maximum encapsulation rates during this time (Krams et al., 2013a, b).
To quantify the encapsulation reaction against the nylon monofilament, we estimated the lightness of each nylon insert after it had been thoroughly dried. This occurs because a parasite or other foreign body is encapsulated with melanin as part of the immune defense mechanism. Specifically, once these invaders are recognized, the pro-phenoloxidase pathway is triggered, activating the phenoloxidase enzyme. This enzymatic activity results in the encapsulation of parasites with melanin (Ratcliffe et al., 1985). Therefore, the melanization of implants is highly correlated with phenoloxidase activity and melanin production (Rantala et al., 2000, 2002, 2007). Implant melanization is positively related to the encapsulation of parasites (Paskewitz and Riehle, 1994) and the ability to resist entomopathogenic fungal diseases (Rantala and Roff, 2007). So, higher levels of melanization, or darkening of the filament, indicates increased immune system activity and an anti-parasitism response (Yourth et al., 2001, 2002).
We photographed the removed implants from three directions under consistent light conditions using a Zeiss Lumar V12 Stereo microscope and Axiocam MRc5 digital recorder to quantify lightness. We then analyzed the digital images using image analysis software (Image J, http://rsbweb.nih.gov/ij/). We marked the area of that portion of the insert that was within the bumblebee’s body, and the program calculated the lightness value (expressed in percentage: the darker the pixels, the higher the percentage). Since increasing melanization indicated a more robust immune response in this study, we calibrated the reflectance of an implant before the insertion to zero level.
To assess how distance from apiaries affected the bumblebee infestation rate, we fitted binary logistic regression, using infestation status as a response value, and distance from the apiary as an independent variable. In addition, the study year was included as a categorical factor. To explore factors affecting reproductive success, we fit two linear models. We set queen cell count and worker cell count as dependent variables and distance, infestation status, and study year as independent variables in each model. Additionally, we accounted for the interaction between distance and infestation status. We also fitted a linear model with encapsulation rate as a response variable using the same independent variables. Significance of each predictor and their interaction was assessed using two-way ANOVA with Type II sums of squares. In all linear models, the assumption of normality of residuals was checked using the Shapiro-Wilk test and QQ diagrams with residuals. The variance of homoscedasticity model was checked using plots of fitted values and standardized residuals. Data analyses were performed in R software (version 4.4.0). Significance was set at 0.05 in all tests. This study did not require ethical permits.
Distance from the apiary significantly affected the infestation probability of bumblebee nests (likelihood ratio (LR) χ2 = 59.80; df = 1; p < 0.001). The effect did not differ between study years (LR χ² = 0.015; df = 1; p = 0.194). As the distance from the apiary increased by 1 m, the likelihood of a bumblebee nest being infested decreased by 0.6% (Odds Ratio = 0.994; 95% confidence interval (CI): 0.988–0.998; Figure 1).
Figure 1. Probability of Aphomia sociella infestation in bumblebee colonies, and its relationship with distance from an apiary (m). The shaded area represents a 95% confidence interval.
Number of new bumblebee queen cells was significantly affected by distance from the apiary (two-way ANOVA: F1,141 = 598.57; p < 0.001), infestation status (F1,141 = 102.46; p < 0.001), and interaction between the two terms (F1,141 = 26.31; p < 0.001). The effect of the study year was not statistically significant (F5,141 = 0.86; p = 0.51). A distance increase in 1 m also increased the number of bumblebee queen cells in healthy colonies by 0.142 (95% CI: 0.130–0.154; Figure 2a) and by 0.08 (95% CI: 0.049–0.118; Figure 2a) in infested colonies. Being infested decreased the queen cell count by 10.64 cells (95% CI: 4.099–17.176; Figure 2a).
Figure 2. Relationship between the distance of bumblebee nests from a honeybee colony (m) and (a) number of young queen cells, (b) number of worker cells, and (c) encapsulation response of worker bees, depending on the status of infestation with Aphomia sociella larvae. Shaded areas represent a 95% confidence interval.
Bumblebee worker cell counts were also significantly affected by distance from the apiary (two-way ANOVA: F1,141 = 2904.29; p < 0.001) and by bumblebee infestation status (F1,141 = 2991.07; p < 0.001). The interaction between distance from apiary and bumblebee infestation status was also significant (F1,141 = 29.15; p < 0.001). The amount of bumblebee worker cells did not differ between the study years (F5,141 = 2.11; p = 0.068). Every 1 m increase in distance from the apiary increased the worker cell count by 0.440 (95% CI: 0.42–0.46; Figure 2b) in healthy bumblebee colonies and by 0.348 (95% CI 0.296–0.4; Figure 2b) in infested bumblebee colonies. Infested bumblebee colonies additionally had 166.78 (95% CI: 156.97–176.59; Figure 2b) fewer worker cells, regardless of distance from the apiary.
Encapsulation response was significantly affected by distance from the apiary (two-way ANOVA: F1,353 = 619.27; p < 0.001) and infestation status of the bumblebee colony (F1,353 = 3146.76; p < 0.001). There was no significant interaction between distance from apiary and bumblebee infestation status (F1,353 = 0.55; p = 0.46). The study year did not impact the encapsulation response (F5,353 = 0.93; p = 0.46). The encapsulation response increased per 1 m of distance by 0.035 (95% CI: 0.031–0.038; Figure 2c), whereas being infested decreased encapsulation rate by 29.8 (95% CI: 28.063–31.540; Figure 2c).
We observed that A. sociella infestation in bumblebee colonies significantly increased with closer proximity to apiaries, where infestation was close to zero at approximately 600 m from apiaries. We also found that reproductive performance, measured by the number of young queen cells and worker cells, was lowest near honeybee apiaries. These results suggest that bumblebee reproductive performance may be influenced by competition with honeybees for similar food resources, as well as influenced by the higher potential for A. sociella infestation in closer proximity to apiaries. Indeed, encapsulation response in bumblebee workers was weakest near apiaries, and the strength of this immune response depends on bumblebee health and infestation status. In other words, the healthiest bumblebee nests, in terms of lowest likelihood for infestation and highest likelihood for greater reproductive performance, were the ones furthest from the apiaries.
There is significant niche overlap and more specifically, similar food preferences, between bumblebees and honeybees, resulting in substantial interspecific competition (Steffan-Dewenter and Tscharntke, 2000; Antúnez et al., 2015; Bernhardsson et al., 2024). However, it is common for honeybees to outcompete bumblebees for these food resources: given that both species are generalists and have similar foraging strategies and radii, the sheer number of individuals in honeybee colonies leads to more total flower visits and overall food consumption, thus decreasing the amount of potential food resources for bumblebees to consume (Goulson and Darvill, 2004). In our system, malnutrition from being outcompeted for food resources likely contributed to a weakened immune response if a colony was infested and lower overall reproductive output. Malnutrition arises as bumblebees disproportionately expend more energy during increased foraging without gaining that energy back from flowers because honeybees have previously exploited that resource (Straub et al., 2023). Malnutrition and lack of energetic resources increases stress, and this cascading effect lowers immunity and reproduction (i.e., fitness). As the immune system requires resources to maintain an organism’s ability to respond to pathogens, competition for limited resources may affect the strength of an organism’s immune responses (Kedia-Mehta and Finlay, 2019). Competition is not restricted to the organismal level, as different arms of immunity can compete for a shared pool of resources under nutrient scarcity, which may increase the risk of impaired reproductive fitness or even death.
The number of young queen cells and worker cells, as well as encapsulation response, were highest away from apiaries. Furthermore, these responses differed significantly between infested and non-infested B. terrestris colonies. We observed weakened immune responses in infested bumblebee colonies compared to that of non-infested colonies at the same distance from apiaries. A. sociella evidently suppressed the immune responses of bumblebees, making these colonies much more susceptible to other pathogens and/or parasites. Since we found A. sociella infestation probability to be strongly associated with proximity to honeybees, and a suppressed encapsulation rate resultant of this immunocompromise, it appears that increased interspecific competition and decreased reproductive output are not the only negative fitness costs incurred by bumblebees when their colonies are near those of honeybees (Meeus et al., 2011). Our study reveals that there are a myriad of factors contributing to decreased fitness in bumblebees near apiaries. The stress and malnutrition from proximity-based interspecific competition likely results in increased susceptibility to infestation and an inability to combat infestation with a strong immune response (Logan et al., 2005). Overall health and reproduction are then depressed in bumblebee colonies, and they may be unable to adequately pollinate specialized plants within their given niche (Williams and Osborne, 2009). Higher numbers of young queen cells and worker cells, and greater encapsulation responses, are important for maintaining sufficient fitness in bumblebee populations. Our results demonstrate that maintaining the parameters of population fitness becomes more difficult when bumblebee colonies are infested with A. sociella. As A. sociella infestation is observed to be greater in bumblebee colonies that are in closer proximity to apiaries, this raises a cascade of concerns regarding honeybee colony placement and its potential impact on the health of wild bumblebee populations (Gillespie, 2010).
Honeybees are undoubtedly a foundational component of plant–pollinator systems, significantly contributing to agricultural production and ecosystem health. Previous studies have demonstrated that landscape characteristics (e.g., vegetation structure and diversity; Herbertsson et al., 2016), overall resource availability (Forup and Memmott, 2005), and spatiotemporal variables (e.g., seasonality; Wignall et al., 2020) influence the degree of competition between honeybees and bumblebees. For instance, in homogeneous agricultural landscapes, niche overlap and competition for food resources are often highest, leading to a significant reduction in bumblebee abundance and body size (Goulson and Sparrow, 2009; Sõber et al., 2020). In contrast, heterogeneous landscapes mitigate these competitive effects by providing increased resource abundance and enabling bumblebees to forage without complete niche overlap (Mänd et al., 2002). Our study, however, introduces the additional variable of parasitic infestation probability. Even if competition is mitigated by landscape characteristics, infestation spillover from introduced apiaries to native bumblebees remains a critical issue. This underscores the importance of considering multiple factors when deciding whether and where to place honeybee colonies. For example, in areas with high local pollinator biodiversity, avoiding apiary placement in such biodiversity hotspots should be prioritized. Finally, future experiments should be designed to confirm the sources of A. sociella infestation, as our field study does not rule out the possibility that local wild bees or wasps could also infest bumblebees in some study sites.
Parasites and pathogens that can impact the health of bumblebees represent a key focus in the health of bumblebee populations in both agricultural operations (e.g., greenhouses) and their natural environments. Indeed, there has long been concern over the potential spread of parasites and pathogens from honeybees to bumblebees (Genersch et al., 2006; Singh et al., 2010; Evison et al., 2012). Our data provide valuable insights on the spatial ecology of A. sociella infestations in areas where honeybees and bumblebees coexist. Our demonstration of reduced immune response in individuals within bumblebee colonies in closer proximity to apiaries is alarming, given that this reduction in immune response could potentially facilitate additional infestations of other parasites and pathogens within bumblebee colonies (e.g., due to a population’s decreased ability to fight off diseases). We recommend conducting more detailed studies on the diversity of parasites and pathogens and their effects on bumblebees in relation to their distance from apiaries. Conservationists must devise plans for introducing honeybees that maximize plant pollination and agricultural output while minimizing negative fitness effects (e.g., potential competitive exclusion, pathogen introduction, decreased reproduction) in native pollinator species.
The datasets presented in this study can be found in online repositories. The names of the repository/repositories and accession number(s) can be found in the article/supplementary material.
The manuscript presents research on animals that do not require ethical approval for their study.
RK: Conceptualization, Data curation, Investigation, Methodology, Writing – review & editing. TG: Investigation, Methodology, Validation, Writing – review & editing. JW: Validation, Writing – original draft, Writing – review & editing. SP: Investigation, Methodology, Writing – review & editing. MM: Formal analysis, Software, Validation, Writing – original draft. GT: Data curation, Formal analysis, Software, Visualization, Writing – review & editing. JC-G: Methodology, Validation, Writing – review & editing. AD: Methodology, Validation, Writing – review & editing. CA: Methodology, Validation, Writing – original draft, Writing – review & editing. MR: Conceptualization, Methodology, Writing – review & editing. SG: Formal analysis, Validation, Writing – review & editing. ES: Formal analysis, Methodology, Writing – review & editing. TK: Conceptualization, Investigation, Methodology, Supervision, Writing – review & editing. IAK: Conceptualization, Methodology, Investigation, Funding acquisition, Supervision, Formal analysis, Writing – original draft, Writing – review & editing.
The author(s) declare that financial support was received for the research, authorship, and/or publication of this article. This publication/research supported within the framework of the European Union’s Recovery and Resilience Mechanism project No.5.2.1.1.i.0/2/24/I/CFLA/001 “Consolidation of the Latvian Institute of Organic Synthesis and the Latvian Biomedical Research and Study Centre” to IAK.
We thank the Fulbright US Student Program, the Latvian Fulbright Post, and the US Department of State.
The authors declare that the research was conducted in the absence of any commercial or financial relationships that could be construed as a potential conflict of interest.
The author(s) declared that they were an editorial board member of Frontiers, at the time of submission. This had no impact on the peer review process and the final decision.
The author(s) declare that no Generative AI was used in the creation of this manuscript.
All claims expressed in this article are solely those of the authors and do not necessarily represent those of their affiliated organizations, or those of the publisher, the editors and the reviewers. Any product that may be evaluated in this article, or claim that may be made by its manufacturer, is not guaranteed or endorsed by the publisher.
Antúnez K., Anido M., Branchiccela B., Harriet J., Campa J., Invernizzi C., et al. (2015). Seasonal variation of honeybee pathogens and its association with pollen diversity in Uruguay. Microbial. Ecol. 70, 522–533. doi: 10.1007/s00248-015-0594-7
Bernhardsson O., Kendall L., Olsson O., Olsson P., Smith H. G. (2024). Shared use of a mass-flowering crop drives dietary niche overlap between managed honeybees and bumblebees. J. Appl. Ecol. 61, 2135–2145. doi: 10.1111/1365-2664.14743
Cardoso P., Barton P. S., Birkhofer K., Chichorro F., Deacon C., Fartmann T., et al. (2020). Scientists’ warning to humanity on insect extinctions. Biol. Conserv. 242, 108426. doi: 10.1016/j.biocon.2020.108426
Chowdhury S., Jennions M. D., Zalucki M. P., Maron M., Watson J. E., Fuller R. A. (2023). Protected areas and the future of insect conservation. Trends Ecol. Evol. 38, 85–95. doi: 10.1016/j.tree.2022.09.004
Dicks L. V., Breeze T. D., Ngo H. T., Senapathi D., An J., Aizen M. A., et al. (2021). A global-scale expert assessment of drivers and risks associated with pollinator decline. Nat. Ecol. Evol. 5, 1453–1461. doi: 10.1038/s41559-021-01534-9
Dubovskii I. M., Grizanova E. V., Chertkova E. A., Slepneva I. A., Komarov D. A., Vorontsova Y. L., et al. (2010). Generation of reactive oxygen species and activity of antioxidants in hemolymph of the moth larvae Galleria mellonella (L.) (Lepidoptera: Piralidae) at development of the process of encapsulation. J. Evolution. Biochem. Physiol. 46, 35–43. doi: 10.1134/S0022093010010044
Elbgami T., Kunin W. E., Hughes W. O., Biesmeijer J. C. (2014). The effect of proximity to a honeybee apiary on bumblebee colony fitness, development, and performance. Apidologie 45, 504–513. doi: 10.1007/s13592-013-0265-y
Evans E., Strange J., Sadd B., Tripodi A., Figueroa L., Adams L., et al. (2023). Parasites, parasitoids, and hive products that are potentially deleterious to wild and commercially raised bumble bees (Bombus spp.) in North America. J. Pollin. Ecol. 33, 37–53. doi: 10.26786/1920-7603(2023)710
Evison S. E., Roberts K. E., Laurenson L., Pietravalle S., Hui J., Biesmeijer J. C., et al. (2012). Pervasiveness of parasites in pollinators. PloS One 7, e30641. doi: 10.1371/journal.pone.0030641
Fontaine C., Dajoz I., Meriguet J., Loreau M. (2006). Functional diversity of plant–pollinator interaction webs enhances the persistence of plant communities. PloS Biol. 4, e1. doi: 10.1371/journal.pbio.0040001
Forup M. L., Memmott J. (2005). The relationship between the abundances of bumblebees and honeybees in a native habitat. Ecol. Entomol. 30, 47–57. doi: 10.1111/j.0307-6946.2005.00660.x
Gambino P. (1995). Dolichovespula (Hymenoptera: Vespidae), hosts of Aphomia sociella (L.) (Lepidoptera: Pyralidae). J. New York Entomol. Soc. 103, 165–169.
Genersch E., Yue C., Fries I., de Miranda J. R. (2006). Detection of deformed wing virus, a honey bee viral pathogen, in bumble bees (Bombus terrestris and Bombus pascuorum) with wing deformities. J. Invertebr. Pathol. 91, 61–63. doi: 10.1016/j.jip.2005.10.002
Gillespie S. (2010). Factors affecting parasite prevalence among wild bumblebees. Ecol. Entomol. 35, 737–747. doi: 10.1111/j.1365-2311.2010.01234.x
Goulson D., Darvill B. (2004). Niche overlap and diet breadth in bumblebees; are rare species more specialized in their choice of flowers? Apidologie 35, 55–63. doi: 10.1051/apido:2003062
Goulson D., Nicholls E., Botías C., Rotheray E. L. (2015). Bee declines driven by combined stress from parasites, pesticides, and lack of flowers. Science 347, 1255957. doi: 10.1126/science.1255957
Goulson D., Sparrow K. R. (2009). Evidence for competition between honeybees and bumblebees; effects on bumblebee worker size. J. Insect Conserv. 13, 177–181. doi: 10.1007/s10841-008-9140-y
Gupta A. P. (1986). Arthropod immunocytes. Identification, structure, functions, and analogies to the functions of vertebrate B-and T-lymphocytes. In Gutpa A. P. (ed): Hemocytic and humoral immunity in Arthropods. New York: John Wiley & Sons, pp. 3–59.
Hallmann C. A., Sorg M., Jongejans E., Siepel H., Hofland N., Schwan H., et al. (2017). More than 75 percent decline over 27 years in total flying insect biomass in protected areas. PloS One 12, e0185809. doi: 10.1371/journal.pone.0185809
Herbertsson L., Lindström S. A., Rundlöf M., Bommarco R., Smith H. G. (2016). Competition between managed honeybees and wild bumblebees depends on landscape context. Basic Appl. Ecol. 17, 609–616. doi: 10.1016/j.baae.2016.05.001
Kedia-Mehta N., Finlay D. K. (2019). Competition for nutrients and its role in controlling immune responses. Nat. Commun. 10, 2123. doi: 10.1038/s41467-019-10015-4
Klein A. M., Vaissière B. E., Cane J. H., Steffan-Dewenter I., Cunningham S. A., Kremen C., et al. (2007). Importance of pollinators in changing landscapes for world crops. Proc. R. Soc. B: Biol. Sci. 274, 303–313. doi: 10.1098/rspb.2006.3721
König C., Schmid-Hempel P. (1995). Foraging activity and immunocompetence in workers of the bumblebee, Bombus terrestris L. Proc. R. Soc. London. Ser. B: Biol. Sci. 260, 225–227. doi: 10.1098/rspb.1995.0084
Kosior A., Celary W., Olejniczak P., Fijał J., Król W., Solarz W., et al. (2007). The decline of the bumble bees and cuckoo bees (Hymenoptera: Apidae: Bombini) of Western and Central Europe. Oryx 41, 79–88. doi: 10.1017/S0030605307001597
Krama T., Krams R., Munkevics M., Willow J., Popovs S., Elferts D., et al. (2022). Physiological stress and higher reproductive success in bumblebees are both associated with intensive agriculture. PeerJ 10, e12953. doi: 10.7717/peerj.12953
Krams I., Daukšte J., Kivleniece I., Kaasik A., Krama T., Freeberg T. M., et al. (2013b). Trade-off between cellular immunity and life span in mealworm beetles Tenebrio molitor. Curr. Zool. 59, 340–346. doi: 10.1093/czoolo/59.3.340
Krams I., Daukste J., Kivleniece I., Krama T., Rantala M. J. (2013a). Previous encapsulation response enhances within individual protection against fungal parasite in the mealworm beetle Tenebrio molitor. Insect Sci. 20, 771–777. doi: 10.1111/j.1744-7917.2012.01574.x
Krams R., Munkevics M., Popovs S., Dobkeviča L., Willow J., Contreras Garduño J., et al. (2021). Ecological stoichiometry of bumblebee castes, sexes, and age groups. Front. Physiol. 12, 696689. doi: 10.3389/fphys.2021.696689
Logan A., Ruiz-González M. X., Brown M. J. F. (2005). The impact of host starvation on parasite development and population dynamics in an intestinal trypanosome parasite of bumble bees. Parasitology 130, 637–642. doi: 10.1017/S0031182005007304
Mänd M., Mänd R., Williams I. H. (2002). Bumblebees in the agricultural landscape of Estonia. Agricult. Ecosys. Environ. 89, 69–76. doi: 10.1016/S0167-8809(01)00319-X
Martins A. C., Silva D. P., De Marco P. Jr., Melo G. A. R. (2015). Species conservation under future climate change: the case of Bombus bellicosus, a potentially threatened South American bumblebee species. J. Insect Conserv. 19, 33–43. doi: 10.1007/s10841-014-9740-7
Meeus I., Brown M. J., De Graaf D. C., Smagghe G. U. Y. (2011). Effects of invasive parasites on bumble bee declines. Conserv. Biol. 25, 662–671. doi: 10.1111/j.1523-1739.2011.01707.x
Ollerton J., Winfree R., Tarrant S. (2011). How many flowering plants are pollinated by animals? Oikos 120, 321–326. doi: 10.1111/j.1600-0706.2010.18644.x
Orr M. C., Hughes A. C., Chesters D., Pickering J., Zhu C. D., Ascher J. S. (2021). Global patterns and drivers of bee distribution. Curr. Biol. 31, 451–458. doi: 10.1016/j.cub.2020.10.053
Page M. L., Williams N. M. (2023). Evidence of exploitative competition between honey bees and native bees in two California landscapes. J. Anim. Ecol. 92, 1802–1814. doi: 10.1111/1365-2656.13973
Paskewitz S., Riehle M. A. (1994). Response of Plasmodium refractory and susceptible strains of Anopheles gambiae to inoculated Sephadex beads. Dev. Comp. Immunol. 18, 369–375. doi: 10.1016/0145-305X(94)90002-7
Plischuk S., Meeus I., Smagghe G., Lange C. E. (2011). Apicystis bombi (Apicomplexa: Neogregarinorida) parasitizing Apis mellifera and Bombus terrestris (Hymenoptera: Apidae) in Argentina. Environ. Microbiol. Rep. 3, 565–568. doi: 10.1111/j.1758-2229.2011.00261.x
Potts S. G., Biesmeijer J. C., Kremen C., Neumann P., Schweiger O., Kunin W. E. (2010). Global pollinator declines: trends, impacts and drivers. Trends Ecol. Evol. 25, 345–353. doi: 10.1016/j.tree.2010.01.007
Potts S. G., Imperatriz-Fonseca V., Ngo H. T., Aizen M. A., Biesmeijer J. C., Breeze T. D., et al. (2016). Safeguarding pollinators and their values to human well-being. Nature 540, 220–229. doi: 10.1038/nature20588
Rantala M. J., Jokinen I., Kortet R., Vainikka A., Suhonen J. (2002). Do pheromones reveal male immunocompetence? Proc. R. Soc. London. Ser. B: Biol. Sci. 269, 1681–1685. doi: 10.1098/rspb.2002.2056
Rantala M. J., KoskimÌki J., Taskinen J., Tynkkynen K., Suhonen J. (2000). Immunocompetence, developmental stability and wingspot size in the damselfly Calopteryx splendens L. Proc. R. Soc. London. Ser. B: Biol. Sci. 267, 2453–2457. doi: 10.1098/rspb.2000.1305
Rantala M. J., Roff D. A. (2007). Inbreeding and extreme outbreeding cause sex differences in immune defence and life history traits in Epirrita autumnata. Heredity 98, 329–336. doi: 10.1038/sj.hdy.6800945
Rantala M. J., Roff D. A., Rantala L. M. (2007). Forceps size and immune function in the earwig Forficula auricularia L. Biol. J. Linn. Soc. 90, 509–516. doi: 10.1111/j.1095-8312.2007.00741.x
Ratcliffe N. A., Rowley A. F., Fitzgerald S. W., Rhodes C. P. (1985). Invertebrate immunity: basic concepts and recent advances. Int. Rev. Cytol. 97, 183–350. doi: 10.1016/S0074-7696(08)62351-7
Raven P. H., Wagner D. L. (2021). Agricultural intensification and climate change are rapidly decreasing insect biodiversity. Proc. Natl. Acad. Sci. 118, e2002548117. doi: 10.1073/pnas.2002548117
Russell A. L., Buchmann S. L., Ascher J. S., Wang Z., Kriebel R., Jolles D. D., et al. (2024). Global patterns and drivers of buzzing bees and poricidal plants. Curr. Biol. 34, 3055–3063. doi: 10.1016/j.cub.2024.05.065
Sadd B. M., Schmid-Hempel P. (2009). Principles of ecological immunology. Evolution. Appl. 2, 113–121. doi: 10.1111/j.1752-4571.2008.00057.x
Salvarrey S., Antúnez K., Arredondo D., Plischuk S., Revainera P., Maggi M., et al. (2021). Parasites and RNA viruses in wild and laboratory reared bumble bees Bombus pauloensis (Hymenoptera: Apidae) from Uruguay. PloS One 16, e0249842. doi: 10.1371/journal.pone.0249842
Schmid-Hempel P. (1998). Parasites in social insects (Princeton, New Jersey: Princeton University Press).
Schmid-Hempel P. (2021). Sociality and parasite transmission. Behavioral Ecology and Sociobiology 75(11):156. doi: 10.1007/s00265-021-03092-3
Schmid-Hempel R., Eckhardt M., Goulson D., Heinzmann D., Lange C., Plischuk S., et al. (2014). The invasion of southern South America by imported bumblebees and associated parasites. J. Anim. Ecol. 83, 823–837. doi: 10.1111/1365-2656.12185
Schweiger O., Biesmeijer J. C., Bommarco R., Hickler T., Hulme P. E., Klotz S., et al. (2010). Multiple stressors on biotic interactions: how climate change and alien species interact to affect pollination. Biol. Rev. 85, 777–795. doi: 10.1111/j.1469-185X.2010.00125.x
Seibold S., Rammer W., Hothorn T., Seidl R., Ulyshen M. D., Lorz J., et al. (2021). The contribution of insects to global forest deadwood decomposition. Nature 597, 77–81. doi: 10.1038/s41586-021-03740-8
Singh R., Levitt A. L., Rajotte E. G., Holmes E. C., Ostiguy N., Vanengelsdorp D., et al. (2010). RNA viruses in hymenopteran pollinators: evidence of inter-taxa virus transmission via pollen and potential impact on non-Apis Hymenopteran species. PloS One 5, e14357. doi: 10.1371/journal.pone.0014357
Sõber V., Leps M., Kaasik A., Mänd M., Teder T. (2020). Forest proximity supports bumblebee species richness and abundance in hemi-boreal agricultural landscape. Agricult. Ecosys. Environ. 298, 106961. doi: 10.1016/j.agee.2020.106961
Steffan-Dewenter I., Tscharntke T. (2000). Resource overlap and possible competition between honey bees and wild bees in central Europe. Oecologia 122, 288–296. doi: 10.1007/s004420050034
Stork N. E. (2018). How many species of insects and other terrestrial arthropods are there on Earth? Annu. Rev. Entomol. 63, 31–45. doi: 10.1146/annurev-ento-020117-043348
Strand M. R. (2008). Insect hemocytes and their role in immunity. Insect Immunol. 32, 25–47. doi: 10.1016/B978-012373976-6.50004-5
Straub F., Birkenbach M., Leonhardt S. D., Ruedenauer F. A., Kuppler J., Wilfert L., et al. (2023). Land-use-associated stressors interact to reduce bumblebee health at the individual and colony level. Proc. R. Soc. B 290, 20231322. doi: 10.1098/rspb.2023.1322
Straub L., Strobl V., Yañez O., Albrecht M., Brown M. J., Neumann P. (2022). Do pesticide and pathogen interactions drive wild bee declines? Int. J. Parasitol.: Parasites Wildl. 18, 232–243. doi: 10.1016/j.ijppaw.2022.06.001
Szabo N. D., Colla S. R., Wagner D. L., Gall L. F., Kerr J. T. (2012). Do pathogen spillover, pesticide use, or habitat loss explain recent North American bumblebee declines? Conserv. Lett. 5, 232–239. doi: 10.1111/j.1755-263X.2012.00234.x
Thomson D. (2004). Competitive interactions between the invasive European honey bee and native bumble bees. Ecology 85, 458–470. doi: 10.1890/02-0626
Thomson D. M. (2006). Detecting the effects of introduced species: a case study of competition between Apis and Bombus. Oikos 114, 407–418. doi: 10.1111/j.2006.0030-1299.14604.x
Turo K. J., Reilly J. R., Fijen T. P., Magrach A., Winfree R. (2024). Insufficient pollinator visitation often limits yield in crop systems worldwide. Nat. Ecol. Evol. 8, 1612–1622. doi: 10.1038/s41559-024-02460-2
Van Klink R., Bowler D. E., Gongalsky K. B., Swengel A. B., Gentile A., Chase J. M. (2020). Meta-analysis reveals declines in terrestrial but increases in freshwater insect abundances. Science 368, 417–420. doi: 10.1126/science.aax9931
Wagner D. L. (2020). Insect declines in the anthropocene. Annu. Rev. Entomol. 65, 457–480. doi: 10.1146/annurev-ento-011019-025151
Wagner D. L., Grames E. M., Forister M. L., Berenbaum M. R., Stopak D. (2021). Insect decline in the Anthropocene: death by a thousand cuts. Proc. Natl. Acad. Sci. 118, e2023989118. doi: 10.1073/pnas.2023989118
Wallin E. A., Kalinová B., Kindl J., Hedenström E., Valterová I. (2020). Stereochemistry of two pheromonal components of the bumblebee wax moth, Aphomia sociella. Sci. Rep. 10, 2094. doi: 10.1038/s41598-020-59069-1
Walther-Hellwig K., Fokul G., Frankl R., Büchler R., Ekschmitt K., Wolters V. (2006). Increased density of honeybee colonies affects foraging bumblebees. Apidologie 37, 517–532. doi: 10.1051/apido:2006035
Wignall V. R., Campbell Harry I., Davies N. L., Kenny S. D., McMinn J. K., Ratnieks F. L. (2020). Seasonal variation in exploitative competition between honeybees and bumblebees. Oecologia 192, 351–361. doi: 10.1007/s00442-019-04576-w
Williams P. H., Osborne J. L. (2009). Bumblebee vulnerability and conservation world-wide. Apidologie 40, 367–387. doi: 10.1051/apido/2009025
Wilson R. J., Fox R. (2021). Insect responses to global change offer signposts for biodiversity and conservation. Ecol. Entomol. 46, 699–717. doi: 10.1111/een.12970
Yourth C. P., Forbes M. R., Smith B. P. (2001). On understanding variation in immune expression of the damselflies Lestes spp. Can. J. Zool. 79, 815–821. doi: 10.1139/z01-044
Keywords: bumblebee, honeybee, immunity, competition, Aphomia sociella infestation
Citation: Krams R, Grigorjeva T, Willow J, Popovs S, Munkevics M, Trakimas G, Contreras-Garduño J, de Souza AR, Adams CB, Rantala MJ, Garajeva SJ, Sledevskis E, Krama T and Krams IA (2025) Infestation levels of Aphomia sociella in bumblebees increase with proximity to apiaries and result in lower reproductive output and weaker immune response. Front. Bee Sci. 3:1550560. doi: 10.3389/frbee.2025.1550560
Received: 23 December 2024; Accepted: 18 February 2025;
Published: 10 April 2025.
Edited by:
Kiyoshi Kimura, Institute of Livestock and Grassland Science (NARO), JapanReviewed by:
Estela Santos, Universidad de la República, UruguayCopyright © 2025 Krams, Grigorjeva, Willow, Popovs, Munkevics, Trakimas, Contreras-Garduño, de Souza, Adams, Rantala, Garajeva, Sledevskis, Krama and Krams. This is an open-access article distributed under the terms of the Creative Commons Attribution License (CC BY). The use, distribution or reproduction in other forums is permitted, provided the original author(s) and the copyright owner(s) are credited and that the original publication in this journal is cited, in accordance with accepted academic practice. No use, distribution or reproduction is permitted which does not comply with these terms.
*Correspondence: Indrikis A. Krams, aW5kcmlraXMua3JhbXNAcHJvdG9uLm1l
Disclaimer: All claims expressed in this article are solely those of the authors and do not necessarily represent those of their affiliated organizations, or those of the publisher, the editors and the reviewers. Any product that may be evaluated in this article or claim that may be made by its manufacturer is not guaranteed or endorsed by the publisher.
Research integrity at Frontiers
Learn more about the work of our research integrity team to safeguard the quality of each article we publish.